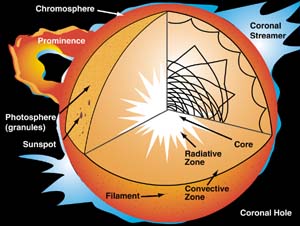
Solar Structure
Courtesy: McREL |
The interior structure of the sun is still somewhat of a
mystery. The opaque mass of the sun is traditionally thought
of as being organized in layers around a central core. From
the core outward, these layers are called: the radiative layer
(or zone), the convection layer, and the photosphere, which
is the surface layer we observe. Surrounding the sun is its
atmosphere, just like the air around the Earth. The lower
layer of the sun's atmosphere is called the chromosphere,
and the outer layer of its atmosphere is the corona.
The Core of the Sun
The extremely dense solar core holds about 50% of
the sun's total mass, but takes up only about 1.5% of its
total volume. Physical conditions inside the sun's core are
extreme. The temperature is thought to be around 15 million
degrees Kelvin, a temperature so extreme that atoms are stripped
of their electrons. Thus the sun's core is a mixture of protons,
neutrons, nuclei, and free electrons.
The pressure at the core is perhaps 250 billion times the
pressure of the atmosphere at the Earth's surface. The sun's
huge mass does not collapse inward by gravitational attraction
only because of this enormous outward pressure generated by
the heat of the core. Also, the sun does not explode like
a hydrogen bomb, because the stupendous mass of the gases
above the core contains its explosiveness.
The density at the sun's core is likewise extremely high.
At the core is the nuclear furnace that produces the sun's
energy. If we could see into the core, it would appear black,
since none of the energy produced there lies in the visible
part of the spectrum. The sun mainly produces short-wavelength
gamma rays. Some of these gamma rays collide with each
other, losing energy. As they lose energy they transform to
x-rays, still not visible to the human eye.

Visible Color Spectrum
The Radiative Layer The
x-rays produced in the sun's core gradually work their way
to the surface of the sun, following a path through bubbles
of reduced temperature, pressure, and density. The radiative
layer extends from the core about 70% of the way up to the
surface. Hydrogen and helium nuclei and unattached electrons
populate the radiative layer. In this layer, some of the loose
electrons are captured by helium nuclei
to form ionized helium atoms .
The radiative layer becomes full of ionized hydrogen
and helium atoms. This mixture of ionized hot gases and electrons
is called plasma and is sometimes regarded as a fourth
state of matter.
Deep in the radiative layer, the x-rays collide with particles
and change direction in random ways. Each x-ray may travel
only a few millimeters before it suffers another collision
and is set off in a different direction. Nevertheless, the
x-rays continue to move toward the surface. The time to complete
this journey to the surface is measured in millions of years,
an incredible fact given that x-rays travel at the speed of
light! To put it in more personal terms, the sunlight that
gave you your most recent sunburn resulted from a nuclear
reaction that took place perhaps 1,000,000 years ago deep
within the core of the sun.
The bumping together of the x-rays in the radiative layer
robs them of their energy. Consequently, their wavelengths
gradually increase as they move upward toward the convection
zone, finally becoming visible light.
The Convection Layer
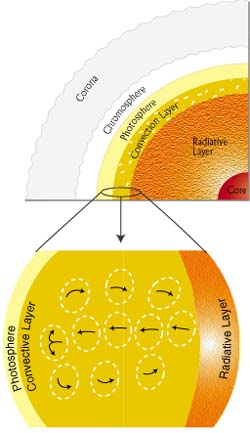
Gas Movement in the Convection Layer
Courtesy: McREL |
Finally, the photons arrive at the convection layer,
150,000 km below the sun's surface, where temperatures are
slightly less than 1 million degrees Kelvin. Here, nuclei
are able to hold onto electrons, and undamaged atoms exist.
With reduced energy, the light is absorbed by gaseous atoms
in the convection zone which hold onto it rather than bouncing
it off or absorbing and then re-radiating it. These atoms
effectively block the outward flow of the sun's energy by
absorbing it and becoming enormously hot. Convection
currents, like those observable in warming liquids and air,
carry the sun's energy to the photosphere on seething rivers
of hot gases.
As the temperature of the gas that has absorbed energy at
the bottom of the convection zone increases, the gas expands,
becoming less dense than its surroundings. These bundles of
hot gas, because they are less dense, float up toward the
surface of the convection layer like hot air balloons through
a cold morning. At the top of this layer, they radiate away
their excess energy, becoming cooler and denser, and then
sinking down again through the convection layer. The effect
is of "conveyor belts" of hot gas moving up and cooler gas
moving down.
At the surface between the convection layer and the photosphere,
the gas is very turbulent, rising up in the center of structures
called convection cells (supergranules), flowing to the cell
boundaries and then sinking. The processes going on at these
cell boundaries, where plasmas with oppositely-oriented magnetic
fields collide and where magnetic energy is converted into
motion, are probably responsible for the heating of the sun's
corona and the acceleration of the solar wind particles which
will be collected by the Genesis spacecraft.
Interestingly, although it may have taken the radiation millions
of years to reach the bottom of the convection zone, its energy
moves up through the entire layer in about three months. All
of the energy sent into space from the surface of the sun
is transported there by convection.
The Photosphere
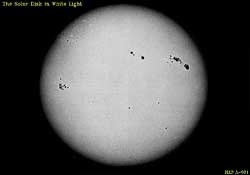
Photosphere with Sunspots
Courtesy: NASA |
Above the convection zone is the photosphere, the visible
bright surface of the sun. Since the photosphere is
a gas, its outer limit is somewhat difficult to define. The
photosphere is probably several hundred kilometers thick.
Temperatures in the photosphere are even lower than in the
convection layer, and the gas densities are quite small (estimated
to be one-millionth the density of water or less). The gaseous
atoms no longer block radiative energy flow. As the hot atoms
cool, they release their excess energy once again as radiation
that streams unimpeded into space and ultimately supports
life on Earth.
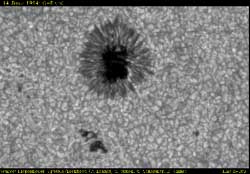
Sunspot Detail
Courtesy: NASA |
The pebbly, granular visible surface of the sun, the photosphere,
is where early astronomers focused most of their attention.
It is here that we find the easily observed blotches that
are now called sunspots. Sunspots come and go in a
regular pattern of about 11 years, but there is uncertainty
about the driving force behind their appearance and disappearance.
They vary in size and often occur in groups that sprawl over
hundreds of millions of square kilometers on the sun's surface.
They look dark because they are cooler than the surrounding
surface of the sun. Sunspots are thought to arise from the
temporary inhibition of convection currents by strong localized
magnetic fields. In other words, if a convection current is
prevented from carrying its load of thermal energy to the
surface, the surface served by that current will cool and
a sunspot will appear. Intervals of high sunspot activity
usually coincide with a wide range of other dramatic solar
events such as coronal mass ejections (CMEs) and flares, which
often disrupt electronic communications, and possibly even
weather patterns, on Earth.
The Chromosphere
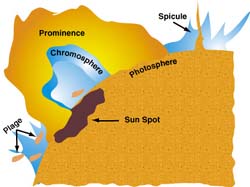
Chromosphere Structures
Courtesy: McREL |
The lower atmosphere of the sunthe chromosphereescaped
scrutiny by early astronomers because it is invisible in contrast
to the bright photosphere below it. The relatively miniscule
amount of light emitted by the chromosphere is only momentarily
visible to the unaided eye during a total solar eclipse when
the moon blocks light from the sun's photosphere. The chromosphere
appears transiently under these conditions as a thin, bright
red ribbon that encircles the silhouette of the moon. Modern
astronomers have been able to study the chromosphere at their
convenience, owing to the wide variety of instruments that
are available to them. Amateur astronomers should never attempt
to view the chromosphere unaided.
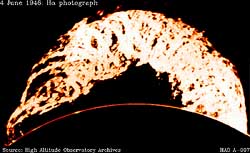
Solar Prominence
Courtesy: NASA |
The chromosphere is an exciting and unique feature of the
solar landscape. Solar astronomers have found there a host
of transient exotic structures, including spicules, prominences,
and plages. The spicules are abundant but short-lived, evanescent
streams of hot gases that vault high into the chromosphere.
More impressive and photogenic are the prominences, some of
which are spectacular bright loops of hot gas that arch high
above the top of the chromosphere and often extend into the
corona. Some of them have widths the size of Earth while others
may approach half the diameter of the sun itself. Prominences
often are associated with sunspots and some of them-the quiescent
prominences- may hold their shape for months before collapsing.
Others-called eruptive prominences-erupt from the chromosphere
as gaseous streamers. Plages are bright, cloud-like structures
that are found in the vicinity of sunspots.
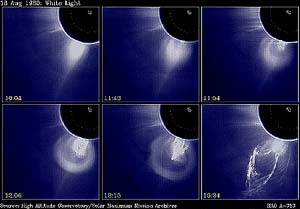
Coronal Mass Ejections (CMEs)
Courtesy: NASA |
Closely related to prominences are monstrously energetic
coronal mass ejections (CMEs) and flares, which typically
begin as a loop that explodes within a few hours and spews
all sorts of solar material into space, including a strong
blast of x-rays and ultraviolet rays. This radiation arrives
at Earth eight minutes later and can severely disrupt the
ionization of the Earth's upper atmosphere. This in turn can
cause major problems with communications and power systems
everywhere on Earth. After about twenty-four minutes, the
next wave hits. This consists of very high-energy protons
that could be extremely harmful to any astronauts who happened
to be in the way. Finally, after one or two days, the Earth
is slammed with a magnetic shock wave travelling at more than
600 miles per second. In 1989, one of the strongest flares
ever observed erupted, causing a power failure all across
the province of Quebec and creating an aurora borealis that
was seen as far south as Key West, Florida.
The power of coronal mass ejections (CMEs) and flares cannot
be overestimated. Flare temperatures may reach 50 million
degrees Kelvin, which is several times hotter than the core
of the sun. If the power of a single CME or flare could be
harnessed, it would be sufficient to provide the energy needs
of the inhabitants of Earth for millions of years.
The chromosphere seems to derive its spectacular behavior
from the dominant force in the solar atmosphere: magnetism.
In contrast to the dense plasmas in the sun's lower regions,
the plasmas of the sun's atmosphere are dilute and unable
to contain the immense magnetic field of the sun. Rather,
the magnetic fields dictate the behavior of the plasma in
the sun's atmosphere, giving rise to the bizarre features
of this region. Loop prominences, for example, are observed
when plasma is captured by magnetic fields and bent back into
the chromosphere.
The Corona
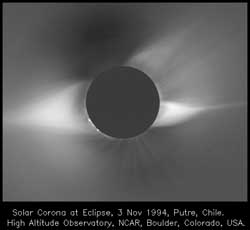
Corona During Eclipse
Courtesy: NASA |
The outermost layer of the solar atmosphere is the corona,
in some ways the most mysterious layer of all. Contrary to
expectations and seemingly to the laws of thermodynamics,
the temperature rises steadily from a minimum of around 4000
degrees Kelvin in the chromosphere to more than a million
degrees Kelvin in the corona. This makes the corona the second
hottest part of the sun, after the sun's core. How is it possible
for heat to be transported from a cooler body (the chromosphere)
to a hotter body (the corona)? Although astrophysicists are
uncertain about the mechanism of energy transfer to the corona,
it is thought by many to be the result of magnetic waves transported
along magnetic field lines emerging from the sun.
The incredibly dilute, superheated gases of the corona reach
millions of miles into space. A total eclipse shows the corona
as a luminous white halo surrounding the glowing disk of the
sun. The corona glows during an eclipse because light from
the photosphere bounces off free electrons in the coronal
plasma. The shape of the corona is synchronized with the solar
activity cycle, changing from a jagged ring around the sun
during the peak of the cycle to wispy plumes and coronal
streamers reaching millions of miles into space at the
end of the cycle. The streamers are the origin of the dense,
lower-speed component of the solar wind.
Mysteries remain about the structure of the sun. The Genesis
mission's study of the solar wind will aid in astrophysicists'
understanding of structures inside the nearest star, the solar
source of Earth's light and heat.
|