Understanding the transition between star and planet
Cosmologists believe that the universe was created about 15 billion years ago with the "big bang", a cosmic explosion which resulted in an expanding cloud of the two lightest elements — the gases hydrogen (H) and helium (He). That's all there was; the Periodic Table back then had only two elements! In some places there were higher concentrations of gas than in others, and the mutual gravitational attractions of the gas molecules in those local concentrations led to the growth of the first generation of stars. As more and more material fell into a new star the pressure at its center finally became high enough to start the process of nuclear fusion in which the nuclei of hydrogen and helium merged to form heavier elements. This was accompanied by the release of energy which made the star begin to shine -- and then there was light!
|
|
|
Eta Carinae: Doomed Star. HST, J. Morse, NASA. |
Eventually all the hydrogen and helium, and those products which could be used to generate energy in the core of the star, were exhausted. Then, the nuclear furnace was extinguished and the outer layers of the star could no longer be supported against gravity. What happened next depended on the mass of the star. In some cases the star became a supernova, exploding violently, rapidly creating even heavier elements, and spewing much of the stellar material into space.
In other cases, the process was slower; instead of an explosion, nuclear reaction products from interior zones were mixed to the surface and then lost to space when the outer layers were blown off. The end results were similar, with the space between the stars being enriched with heavy elements, many of which condensed to form small solid grains. These processes happened over and over again, with each successive generation of stars starting off with higher abundances of heavy elements than the previous generation.
Our Sun belongs to the generation of stars created 4.6 billion years ago, when our galaxy was roughly half its present age. A cloud of interstellar gas, dust, and ices containing several generations of material collapsed to form the nebula from which the Sun and the rest of the solar system grew. This collapse may have been triggered by a nearby supernova.
|
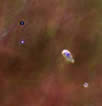 |
|
Protoplanetary disks surrounding stars in the Orion Nebula. HST, Bally, Devine, Sutherland, NASA. |
Because the nebula had some rotational motion, it was not possible for all the nebular material to fall directly into the central mass which would become the Sun. The conservation of angular momentum confined some of the material to a flat, spinning disk around the young Sun. As time went on, the grains and ices in that disk bumped into and stuck to one another. As they grew larger, their gravitational forces increased, attracting more matter from the disk and gradually building up kilometer-sized bodies called planetesimals, some of which in turn formed the nuclei of the planets as we know them today. Other planetesimals either became comets or asteroids.
The nebula is thus the transition state between discrete artifacts of our stellar ancestors and the planetary objects which evolved from it. But how did this evolution from solar nebula to the planets take place? What were the processes that resulted in the birth of current planets and other bodies in our solar system? Partial answers are available from the elemental and isotopic composition of the bodies in the solar system. It appears that every moon and every planet, and even the asteroids are different in elemental and some isotopic compositions.
These differences represent fossil residues which provide invaluable and unique insight into how the solar nebula evolved to form the planets.
In general, one expects that the elemental compositions of planetary objects formed from the solar nebula would be different, reflecting, for example, different distances (temperatures) from the Sun. These differences compared to the average nebula composition allow scientists to model specific formation processes and conditions for a given object.
The current theories, models and assumptions used by planetary scientists attribute these differences to a set of processes that over time resulted in spatial inhomogeneities in the solar nebula, from which the various planets formed. Are these theories and assumptions about the evolution of the solar system accurate? In answering that question, we are handicapped because we do not have a definitive set of initial conditions, i.e., the average isotopic and elemental composition of the original solar nebula. This is a cornerstone data set from which theories about the evolution of the planets begin. A definitive data set would allow us to iterate and refine the current models with these initial conditions until they result in outputs which are consistent with our observations of the isotopic and elemental differences in various planetary bodies.
|
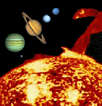 |
|
Scaled images of
the Sun and the
largest planets
(Earth shown as a
dot). More than 99%
of all the material in
the solar system resides in the Sun. |
But how do we get our hands on the average isotopic and elemental composition of the solar system of 4.6 billion years ago? Fortunately for us, this information is largely preserved in the surface layers of the Sun.
The Sun contains most of the mass of the original nebula, and while nuclear reaction has modified the composition at the core, the surface layers which do not mix with the core have preserved the original nebula composition.
We can not send a spacecraft to the Sun to pick up samples for analysis, but the solar matter comes to us in the form of the solar wind. The solar wind is a steady stream of energetic ionized atomic particles which have acquired sufficient kinetic energy to escape the Sun. Solar wind particles, traveling at 300-800 km/s (or about a million miles/hour) are prevented from striking the Earth's atmosphere by the Earth's magnetic field. But all we need to do is to get outside the Earth's magnetosphere, and we have solar matter to sample directly.
The concept of the Genesis mission is to send a spacecraft beyond the influence of Earth to collect pristine material from the solar wind for a period of two years, and then return the samples to Earth for analysis of its elemental and isotopic abundances in state-of-the-art laboratories. These studies will enrich our understanding of the birth and evolution of the planets, their satellites, and all other bodies in our solar system. The Genesis mission will return a reservoir of solar system matter which will be available for study throughout the 21st century -- that is, the samples will endure long after the end of the mission.
|