by Bob Silberg, Science Writer
"It is, of course, a trifle,
but there is nothing
so important as trifles."
— Sherlock Holmes
The Man with the Twisted Lip
by Arthur Conan Doyle
Your last drink of water contained isotopes that could help a Crime Scene Investigation unit determine where you've been living if they find a few strands of your hair. If you're into counterfeiting, you might hear a knock on the door from Secret Service agents who tracked the isotopes in the paper you've been using.
Apollo astronauts brought home lunar rocks containing isotopes that strongly support the theory that the material that makes up the Moon splashed out of the Earth during a collision with a Mars-size planet eons ago. And Genesis scientists are hard at work determining the isotopic composition of the Sun to help solve the puzzle of how the planets began to form out of a cloud of gas and dust.
What are isotopes? They're two or more forms of the same element that contain different numbers of neutrons. In any given atom, the number of protons determines which element it is, and the number of neutrons determines which isotope of that element.
Here's the simplest example:
On the left is an ordinary hydrogen atom, with a single proton as its nucleus. On the right is an isotope of hydrogen known as deuterium. The only difference is that deuterium has a neutron in addition to the proton. The water in your drinking glass, food, and ultimately your body contains both forms of hydrogen in a proportion characteristic of where you live (or at least, where your water comes from).
That water also contains all three stable isotopes of oxygen. Oxygen isotopes are particularly important to Genesis scientists, so let's take a look at them:
Isotope |
Symbol |
Protons |
Neutrons |
% on Earth |
Oxygen-16 |
16O |
8 |
8 |
99.63% |
Oxygen-17 |
17O |
8 |
9 |
0.04% |
Oxygen-18 |
18O |
8 |
10 |
0.20% |
You might notice that the name of each isotope comes from adding the numbers of protons and neutrons, which also tells us its mass (an atom gets one mass unit for each proton and neutron). So oxygen-18 has the most mass, followed by oxygen-17 and then oxygen-16 (far and away the most common oxygen isotope).
Some isotopes are stable, while others are not. The unstable ones are radioactive and change into different elements by turning a neutron into a proton or vice versa, or by ejecting two protons and two neutrons at once (this is called an alpha particle and is the same as the nucleus of a helium atom).
The stable isotopes of any given element behave almost identically in chemical reactions. Both hydrogen isotopes, for example, can hook up with another hydrogen atom (either kind) and an oxygen atom (any of the 3 kinds) to form a molecule of water.
What makes isotopes useful to detectives and planetary scientists is that they tend to redistribute themselves into different proportions in response to various processes.
Mass-dependent fractionation
Evaporation, condensation, biological processes (like photosynthesis), chemical interactions and other influences tend to separate the isotopes by their masses and redistribute them in characteristic ways. Scientists call this redistribution "fractionation."
Water molecules containing lighter isotopes of hydrogen and oxygen, for example, tend to evaporate more readily than molecules containing the heavier, more massive isotopes. Water containing the heavier isotopes tend to condense out more readily than water with the lighter isotopes. Yes, we're only talking about the minuscule mass of a neutron or two, but it makes a difference.
As a result, water vapor tends to have a higher proportion of lighter (16O) to heavier (17O and 18O) oxygen isotopes than the body of water from which it evaporated. Raindrops tend to have a lower proportion of lighter to heavier isotopes than the clouds from which they fell. As rain clouds move from the oceans inland, they become progressively depleted in those heavier isotopes, and the proportion of lighter isotopes increases. So rain falling in the middle of a continent tends to have a higher proportion of lighter isotopes than rain falling along the coasts, and the streams, rivers, and groundwater in those areas reflect those differences.
Other, similar processes tend to redistribute the isotopes of many other elements according to their masses in similarly well-understood ways. Law-enforcement agencies, as well as environmental and other organizations, can take advantage of this phenomenon to identify, at least roughly, where bits of evidence came from.
Non-mass-dependent fractionation
In much the same way that detectives can use isotopic ratios to identify where on Earth a hair, a sheet of paper, or the lead in a bullet came from, scientists can use isotopic ratios to identify whether a particular rock came from Earth, Mars, or an asteroid. In fact, isotopic differences from planet to planet—or from the Sun to the planets—are much more distinct. As Kevin McKeegan, who heads the Genesis mission's UCLA team, said, "You could not confuse a rock from Earth with a rock from Mars. If you could measure its oxygen isotopes, you'd know which one it came from." But the processes responsible for this fractionation are not well-understood.
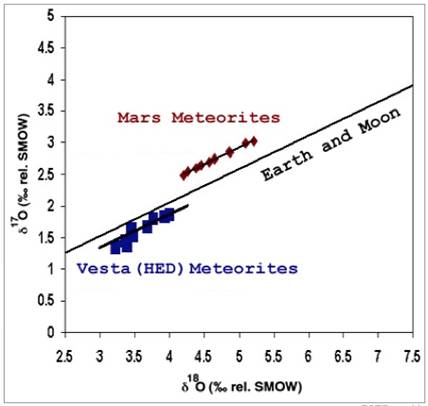 PSRD Graphic
Comparison of oxygen isotopic ratios on Mars, Earth, and meteorites thought to come from Vesta. The Greek letter that precedes 17O and 18O is a lower-case delta. It indicates how much the ratio of each of these isotopes to 16O differs from that same ratio in a reference standard (SMOW), expressed in parts-per-thousand (per mil).
As mentioned, the ratio of oxygen isotopes in different samples on Earth vary in a way that depends on their masses. These ratios are usually expressed in terms of a reference standard called Standard Mean Ocean Water (SMOW) and is typically given as "per mil" instead of "percent." (Per mil means per thousand.) In any given sample on Earth, if the ratio of 18O to 16O deviates from the standard by, for example, 2 per mil, then the ratio of 17O to 16O in that sample will deviate from the standard by about half that amount—in this case, 1 per mil.
If you plot the ratios of various samples on Earth and the Moon, you will get a line like the one shown above, which has a slope of about one-half because the ratio of 17O to 16O always deviates from the standard by about one-half the amount that 18O to 16O deviates from the standard. And that, in turn, is because the difference in the masses of 17O and 16O (namely one) is one-half of the difference in the masses of 18O and 16O (namely two).
Different samples on Mars relate to each other in the same way, based on studies of meteorites believed to have originated on that planet. So do different samples from meteorites thought to have come from an asteroid called Vesta. But Mars appears to have slightly more of the heavier isotopes in proportion to 16O than does Earth, while Vesta appears to have slightly less, so the slope-one-half lines of these three worlds are parallel, with Mars above Earth and Vesta below Earth on the above graph.
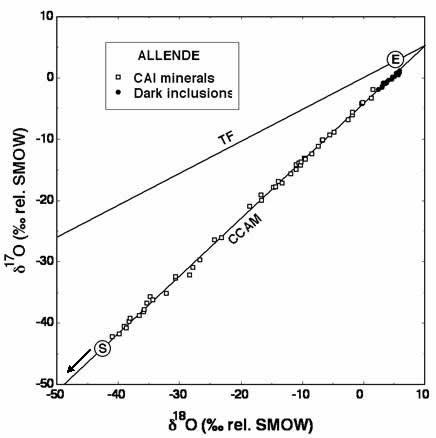
Graph courtesy of Robert Clayton
Comparison of oxygen isotopic ratios on Earth with those of CAIs. "TF" stands for the "terrestrial fractionation" line, the slope-one-half line on which Earthly samples plot. The "E" in a circle indicates approximately where on that line most Earthly samples would be found. The other line is a slope-one line. The open diamonds indicate the isotopic ratios of oxygen in the CAIs of the Allende meteorite. The "S" in a circle represents the approximate area where oxygen isotopes on the Sun fall, based on preliminary results of Genesis Mission studies.
Cosmochemist Robert Clayton and his colleagues caused quite a stir among planetary scientists in 1973 when they analyzed the oxygen isotope ratios of a group of little nuggets (called Calcium-Aluminum-rich Inclusions or CAIs) embedded in the Allende meteorite and found two surprises. First, they contained much less of the heavier isotopes in proportion to 16O than Earth, Mars, or Vesta. And second, the ratios in these CAIs were not distributed along a slope-one-half line. Instead, they varied along a slope-one line—that is, if the ratio of 18O to 16O deviates from the standard by a certain amount, then the ratio of 17O to 16O will deviate from the standard by the same amount. Thus, the effect of whatever process caused this particular distribution of oxygen isotopes in the CAIs does not depend on the masses of the isotopes.
The Allende meteorite's CAIs were found to be the oldest known solids in the solar system. In fact, scientists think they represent the first solids to form out of the solar nebula, the giant cloud of gas and dust from which the entire solar system developed.
Preliminary results from the Genesis mission indicate that the oxygen isotope ratios of the Sun are similar to those of the CAIs, which suggests that the solar nebula had similar ratios as well.
Assuming that these findings are backed up by the analyses remaining to be done, this means that scientists will have both a starting value (that of the ancient solar nebula as represented by the Sun) and a current value (that of the planetary bodies in today's inner solar system) for these ratios. If they can explain the change from one to the other, they may be well on their way to explaining how the planets began to form—the big question behind the Genesis mission.
Four ideas
No one knows for sure what processes are responsible for the change from the oxygen isotope ratios in the original solar nebula to that of the planetary bodies we see today or the differences from planet to planet, but scientists attempting to provide at least a partial explanation have advanced several hypotheses.
One set of models depends not on mass but on the relative abundances of the three isotopes, and attributes the fractionation to a process called "photochemical self-shielding." It's based on the fact that ultraviolet light can break apart molecules of carbon monoxide (CO) into separate carbon and oxygen atoms, and that slightly different wavelengths of ultraviolet light would be required to break up molecules made with each of the three different oxygen isotopes. One wavelength would work on CO made with 16O, a slightly different wavelength would work on CO made with 17O, and a third wavelength would work on CO made with 18O.
Scientists believe that most of the oxygen in the solar nebula was bound up in molecules of CO and water. The idea behind the photochemical self-shielding models is that as ultraviolet light, from stars or from the newly formed Sun, hit the nearest portion of the solar nebula, most of the light tuned to the plentiful 16O isotope would have been absorbed by nearby CO containing that isotope, and little light of that wavelength would have been left to penetrate deeper into the cloud. Thus, CO containing 16O would have remained intact in the interior of the cloud.
On the other hand, because there was so much less 17O and 18O, little of the light tuned to those isotopes would have been absorbed close to the UV source, and much more of the light would have been able to penetrate deep into the cloud, where it would have broken apart CO molecules made with those isotopes. On the whole, a greater percentage of the rarer heavy isotopes than of the common light isotopes would have been liberated from their CO molecules. Those free isotopes would very quickly have bound with other atoms to form new molecules that would ultimately have become the rocks and other ingredients of planets, and the balance of heavier to lighter isotopes in those objects would have been greater than in the CO.
There are currently three versions of this model, which differ according to where and when they say this process took place. One says that the source of the UV light was our young sun, and that it irradiated the inner edge of the cloud surrounding it. Another says that the source was one or more nearby stars, which irradiated the outer regions of the solar nebula. And a third model says that the process predates the formation of the solar nebula, and occurred in the larger molecular cloud from which the nebula formed.
A different hypothesis proposes that the oxygen fractionation in the early solar system depended not on mass or abundance, but rather on the ability of asymmetric molecules to undergo chemical reactions more readily than symmetric molecules. This process has been observed in the production of ozone in Earth's stratosphere among other chemical reactions, and some scientists think a similar process may explain the peculiarities observed in oxygen isotopic ratios in the solar system.
~~~ |