Chapter 7: Mission Inception
During the design and development phase, schedules are negotiated, and the space flight system is designed and developed. The phase begins with building and integrating subsystems and experiments into a single spacecraft. In a process called ATLO (Assembly, Test, and Launch Operations) the spacecraft is assembled integrated, tested, launched, deployed, and verified. Prior to launch, though, the complete spacecraft is tested together in a simulated interplanetary space environment. Voyager, Ulysses, Galileo, Cassini, and many more spacecraft have undergone extensive testing in JPL's 25-foot diameter solar-thermal-vacuum chamber.
Ground systems to support the mission are also developed in parallel with the spacecraft development, and are exercised along with the spacecraft during tests. Phase C/D typically lasts until 30 days after launch. Reviews commonly conducted as part of the design and development phase include: Preliminary Design Review, Critical Design Review, Test Readiness Review, and Flight Readiness Review.
Operations phase E is also called MO&DA for Mission Operations and Data Analysis. It includes flying the spacecraft and obtaining science data for which the mission was designed. This phase is described in later sections of this training module: Chapters 14 through 17 present details of Launch, Cruise, Encounter, Extended Operations, and Project Closeout.
The process by which a mission is conceived and brought through the phases described above includes consideration of many variables. The remainder of this chapter simply touches upon a few of them.
Trajectories are constrained by the laws of celestial mechanics, but the realities of budgets constrain the desires and needs of project science to determine the final choices. Should the mission use a quick, direct path that can be achieved only with a massive upper stage, or an extended cruise with gravity assists for "free" acceleration? Can significant science be accomplished by going only a few weeks out of the way? Which options can be justified against the cost in personnel and time? This task of balancing the monetary, the political and the physical is ordinarily resolved before most project personnel are assigned. The Cassini mission underwent a design change early in its life cycle, reducing its hardware complexity and saving substantial cost initially (although simplifying the hardware did increase the mission's later operational costs somewhat).
The purpose, scope, timing and probable budget for a mission must be clearly understood before realistic spacecraft design can be undertaken. But even a final, approved and funded design may be altered when assumed conditions change during its lifetime. Late design changes are always costly. The Galileo mission design, for example, underwent many significant and costly changes before it was finally launched. The Space Station Freedom spent tens of billions of dollars over several years prior to having comprehensive design changes imposed and becoming the International Space Station.
Timing for many JPL missions is affected most directly by solar system geometry, which dictates optimum launch periods. It correspondingly implies the "part of the sky" that the proposed spacecraft will occupy and how many other spacecraft it may have to compete with for DSN antenna time. If possible, it is very advantageous to fly a mission toward an area where the spacecraft will share little or none of its viewperiod with other missions. Viewperiod is the span of time during which one DSN station can observe a particular spacecraft above its local horizon -- perhaps eight to twelve hours each day.
Years before launch, mission designers request a "what-if" study by JPL's Resource Analysis Team to determine the probable degree of contention for DSN tracking time during the mission. Such a study can assist project management in the selection of launch date and mission profile with the least contention for external resources, and maximized science return for the mission.
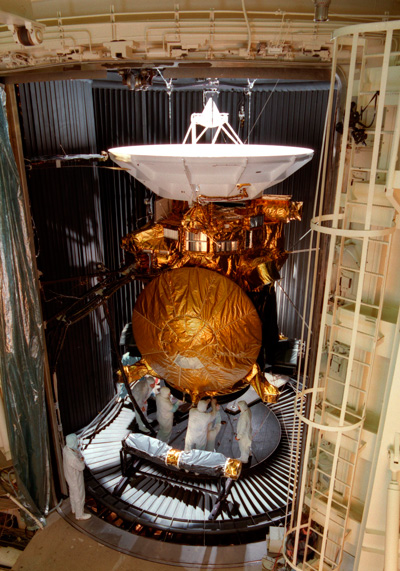
When spacecraft occupy different areas of the sky, as in the April 1993 example in the diagram, contention is at a minimum. However, when several spacecraft are bunched together in the same part of the sky, as they are in the December 1993 example, contention for DSN resources within heavily populated bunches may be formidable. Diagrams such as these are produced by the Resource Allocation Team for 10 year periods. They represent the situation on the 15th day of the month shown. The arrow indicates the center of a spacecraft view from Earth. Extend 60 degrees on both sides of an arrow to describe an 8-hour view period for a spacecraft.
DSN tracking and data handling capabilities must be considered when designing on-board storage, telemetry rates, trajectory and launch periods. Magellan, for example, acquired radar data at 800 kilobits per second. Since it used its high-gain antenna for both mapping and high rate communications, it required on-board storage sufficient to record its data during each mapping pass. The project needed assurance that it could count on DSN tracking time nearly 24 hours a day for the duration of the mission. The data for each orbit had to be downlinked immediately after being acquired or it would be lost, overwritten by data from the next orbit. This scheme made good use of the highly elliptical orbit that Magellan occupied during mapping phase. High-rate data acquisition took place during the 20 or 30 minutes near periapsis, and the hour-long outbound and inbound legs of each orbit were necessary to transmit the data to Earth at the lower rate of 268.8 kbps.
The Mars Global Surveyor spacecraft also has limited on-board storage that requires carefully planned DSN tracking frequency to avoid data loss. The high transmission rate and the maximum distance to Mars must be taken into account when designers determine such things as transmitter power and high-gain antenna size.
Most planetary orbiters, including Galileo and Cassini, face similar tracking and data delivery constraints.
The proposed volume and complexity of the mission's telemetry influences the cost of ground processing. If telemetry does not present significant differences from recent missions, it may be economical to use an adaptation of the existing Advanced Multimission Operations System (AMMOS) rather than develop one that is mission-specific.
In 1985 the Infrared Astronomy Satellite (IRAS) mission's data requirements drove the implementation of an entire data processing facility on the Caltech campus. Known as the Infrared Processing and Analysis Center (IPAC), it is now being used to support the Space Infrared Telescope Facility (Spitzer) mission, the Kepler mission, and many more.
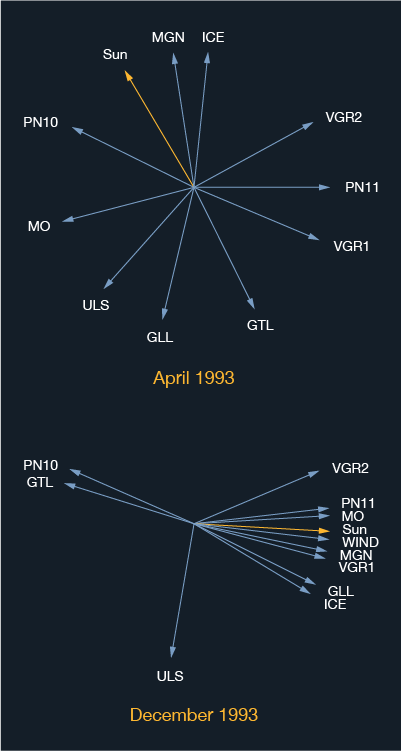
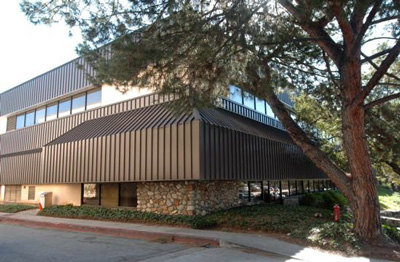